================ Start Lecture #11
================
4.4.7: Simulating (Approximating) LRU in Software
The Not Frequently Used (NFU) PRA
- Include a counter in each PTE (and have R in each PTE).
- Set counter to zero when page is brought into memory.
- For each PTE, every k clock ticks.
- Add R to counter.
- Clear R.
- Choose as victim the PTE with lowest count.
R | counter |
---|
1 | 10000000 |
|
0 | 01000000 |
|
1 | 10100000 |
|
1 | 11010000 |
|
0 | 01101000 |
|
0 | 00110100 |
|
1 | 10011010 |
|
1 | 11001101 |
|
0 | 01100110 |
|
The Aging PRA
NFU doesn't distinguish between old references and recent ones. The
following modification does distinguish.
- Include a counter in each PTE (and have R in each PTE).
- Set counter to zero when page is brought into memory.
- For each PTE, every k clock ticks.
- Shift counter right one bit.
- Insert R as new high order bit (HOB).
- Clear R.
- Choose as victim the PTE with lowest count.
Homework: 25, 34
4.4.8: The Working Set Page Replacement Problem (Peter Denning)
The working set policy (Peter Denning)
The goal is to specify which pages a given process needs to have
memory resident in order for the give process to run without too many
page faults.
- But this is impossible since it requires predicting the future.
- So we make the assumption that the immediate future is well
approximated by the immediate past.
- Measure time in units of memory references, so t=1045 means the time
when the 1045th memory reference is issued.
- In fact we measure time separately
for each process, so t=1045 really means the time when this process
made its 1045th memory reference.
- W(t,&omega) is the set of pages referenced (by the given process) from
time t-ω to time t.
- That is, W(t,ω) is the set pages referenced during
the window of size ω ending at time t.
- That is, W(t,ω) is the set of pages referenced by the last
ω memory references ending at reference t.
- W(t,ω) is called the working set at time t
(with window ω).
- Does this Netscape support the ω notation to give the Greek letter?
- w(t,ω) is the size of the set W(t,ω), i.e. is the
number of pages referenced in the window.
The idea of the working set policy is to ensure that each process
keeps its working set in memory.
- Allocate w(t,ω) frames to each process.
This number differs for each process and changes with time.
- On a fault, one replaces a page not in the working set. But it is
not easy to find such a page quickly.
- Indeed determining W(t,ω) is difficult.
- We will see that the working set algorithm is essentially a
``global policy'' (defined below). I would actually prefer
covering the working set policy after defining local and global
policies but decided to follow Tannenbaum.
Interesting questions include:
- What value should be used for ω?
Experiments have been done and ω is surprisingly robust (i.e.,
for a given system a fixed value works reasonably for a wide variety
of job mixes)
- How should we calculate W(t,ω)?
Hard so do exactly so ...
... Various approximations to the working set, have been devised.
4.4.9: The WSClock Page Replacement Algorithm
- Use the aging algorithm above to maintain a counter for
each PTE and declare a page whose counter is above a certain threshold
to be part of the working set.
- Apply the clock algorithm globally (i.e. to all pages) but
refuse to page out any page in a working set, the resulting algorithm
is called wsclock.
- What if we find there are no pages we can page out?
Simple answer: Pick some page (almost at random).
Another answer: Reduce the multiprogramming level
(explained in 4.6 below).
- Page Fault Frequency (PFF): Described in 4.6 below.
4.4.10: Summary of Page Replacement Algorithms
Algorithm | Comment
|
---|
Random | Poor, used for comparison
|
Optimal | Unimplementable, use for comparison
|
LIFO | Horrible, useless
|
NRU | Crude
|
FIFO | Not good ignores frequency of use
|
Second Chance | Improvement over FIFO
|
Clock | Better (natural) implementation of Second Chance
|
LRU | Great but impractical
|
NFU | Crude LRU approximation
|
Aging | Better LRU approximation
|
Working Set | Good, but expensive
|
WSClock | Good approximation to working set
|
4.5: Modeling Paging Algorithms
4.5.1: Belady's anomaly
Consider a system that has no pages loaded and that uses the FIFO
PRU.
Consider the following ``reference string'' (sequences of pages referenced).
0 1 2 3 0 1 4 0 1 2 3 4
If we have 3 frames this generates 9 page faults (do it).
If we have 4 frames this generates 10 page faults (do it).
Theory has been developed and certain PRA (so called ``stack
algorithms'') cannot suffer this anomaly for any reference string.
FIFO is clearly not a stack algorithm. LRU is. Tannenbaum has a few
details, but we are skipping it.
Repeat the above calculations for LRU.
4.6: Design issues for (demand) Paging Systems
4.6.1: Local vs Global Allocation Policies
A local PRA is one is which a victim page is chosen
among the pages of the same process that requires a new page. That is
the number of pages for each process is fixed. So LRU means the page
least recently used by this process.
- Of course we can't have a purely local policy, why?
Answer: A new process has no pages and even if we didn't apply this for
the first page loaded, the process would remain with only one page.
- Perhaps wait until a process has been running a while or give
the process an initial allocation based on the size of the executable.
- A global policy is one in which the choice of
victim is made among all pages of all processes.
If we apply global LRU indiscriminately with some sort of RR processor
scheduling policy, and memory is somewhat over-committed, then by the
time we get around to a process, all the others have run and have
probably paged out this process.
If this happens each process will need to page fault at a high
rate; this is called thrashing.
It is therefore important to get a good
idea of how many pages a process needs, so that we can balance the
local and global desires. The working set W(t,ω) is good for
this.
An approximation to the working set policy that is useful for
determining how many frames a process needs (but not which pages)
is the Page Fault Frequency (PFF) algorithm.
- For each process keep track of the page fault frequency, which
is the number of faults divided by the number of references.
- Actually, must use a window or a weighted calculation since
you are really interested in the recent page fault frequency.
- If the PFF is too high, allocate more frames to this process.
Either
- Raise its number of frames and use a local policy; or
- Bar its frames from eviction (for a while) and use a
global policy.
- What if there are not enough frames?
Answer: Reduce the MPL (see next section).
As mentioned above a question arises what to do if the sum of the
working set sizes exceeds the amount of physical memory available.
This question is similar to the final point about PFF and brings us to
consider controlling the load (or memory pressure).
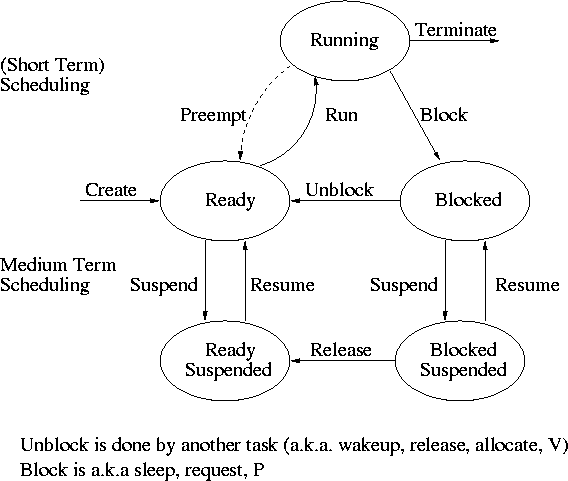
4.6.2: Load Control
To reduce the overall memory pressure, we must reduce the
multiprogramming level (or install more memory while the system is
running, which is hardly practical). That is, we have a
connection between memory management and process management. This is
the suspend/resume arcs we saw way back when.
4.6.3: Page size
- Page size ``must'' be a multiple of the disk block size. Why?
Answer: When copying out a page if you have a partial disk block, you
must do a read/modify/write (i.e., 2 I/Os).
- Important property of I/O that we will learn later this term is
that eight I/Os each 1KB takes considerably longer than one 8KB I/O
- Characteristics of a large page size.
- Good for user I/O.
- If I/O done using physical addresses, then I/O crossing a
page boundary is not contiguous and hence requires multiple
I/Os
- If I/O uses virtual addresses, then page size doesn't effect
this aspect of I/O. That is the addresses are contiguous
in virtual address and hence one I/O is done.
- Good for demand paging I/O.
- Better to swap in/out one big page than several small
pages.
- But if page is too big you will be swapping in data that is
really not local and hence might well not be used.
- Large internal fragmentation (1/2 page size).
- Small page table.
- A very large page size leads to very few pages. Process will
have many faults if using demand
paging and the process frequently references more regions than
frames.
- A small page size has the opposite characteristics.
4.6.4: Separate Instruction and Data (I and D) Spaces
Skipped.
4.6.5: Shared pages
Really should share segments.
- Must keep reference counts or something so that when a process
terminates, pages (even dirty pages) it shares with another process
are not automatically discarded.
- Similarly, a reference count would make a widely shared page (correctly)
look like a poor choice for a victim.
- A good place to store the reference count would be in a structure
pointed to by both PTEs. If stored in the PTEs, must keep them
consistent between processes.
4.6.6: Cleaning Policy (Paging Daemons)
Done earlier
4.6.7: Virtual Memory Interface
Skipped.
4.7: Implementation Issues
4.7.1: Operating System Involvement with Paging
4.7.2: Page Fault Handling
What happens when a process, say process A, gets a page fault?
- The hardware detects the fault and traps to the kernel (switches
to supervisor mode and saves state).
- Some assembly language code save more state, establishes the
C-language (or another programming language) environment, and
``calls'' the OS.
- The OS determines that a page fault occurred and which page was
referenced.
- If the virtual address is invalid, process A is killed.
If the virtual address is valid, the OS must find a free frame.
If there is no free frames, the OS selects a victim frame.
Call the process owning the victim frame, process B.
(If the page replacement algorithm is local process B is process A.)
- If the victim frame is dirty, the OS schedules an I/O write to
copy the frame to disk.
Thus, if the victim frame is dirty, process B is
blocked (it might already be blocked for some other reason).
Process A is also blocked since it needs to wait for this frame to be free.
The process scheduler is invoked to perform a context switch.
- Tanenbaum ``forgot'' some here.
- The process selected by the scheduler (say process C) runs.
- Perhaps C is preempted for D or perhaps C blocks and D runs
and then perhaps D is blocked and E runs, etc.
- When the I/O to write the victim frame completes, a Disk
interrupt occurs. Assume processes C is running at the time.
- Hardware trap / assembly code / OS determines I/O done.
- Processes B is moved from blocked to ready
(unless B is also blocked for some other reason).
- The scheduler picks a process to run, maybe A, maybe B, maybe
C, maybe another processes.
- At some point the scheduler does pick process A to run.
Recall that at this point A is still executing OS code.
- Now the O/S has a clean frame (this may be much later in wall clock
time if a victim frame had to be written).
The O/S schedules an I/O to read the desired page into this clean
frame.
Process A is blocked (perhaps for the second time) and hence the
process scheduler is invoked to perform a context switch.
- A Disk interrupt occurs when the I/O completes (trap / asm / OS
determines I/O done). The PTE is updated.
- The O/S may need to fix up process A (e.g. reset the program
counter to re-execute the instruction that caused the page fault).
- Process A is placed on the ready list and eventually is chosen by
the scheduler to run.
Recall that process A is executing O/S code.
- The OS returns to the first assembly language routine.
- The assembly language routine restores registers, etc. and
``returns'' to user mode.
Process A is unaware that all this happened.
4.7.3: Instruction Backup
A cute horror story. The 68000 was so bad in this regard that
early demand paging systems for the 68000, used two processors one
running one instruction behind. If the first got a page fault, there
wasn't always enough information to figure out what to do so the
system switched to the second processor after it did the page fault.
Don't worry about instruction backup. Very machine dependent and
modern implementations tend to get it right. The next generation
machine, 68010, provided extra information on the stack so the
horrible 2-processor kludge was no longer necessary.
4.7.4: Locking (Pinning) Pages in Memory
We discussed pinning jobs already. The
same (mostly I/O) considerations apply to pages.
4.7.5: Backing Store
The issue is where on disk do we put pages.
- For program text, which is presumably read only, a good choice is
the file itself.
- What if we decide to keep the data and stack each contiguous on
the backing store.
Data and stack grow so must be prepared to grow the space on
disk and leads to the same issues and problems as we saw with MVT.
- If those issues/problems are painful, we can scatter the pages on
the disk.
- That is we employ paging!
- This is NOT demand paging.
- Need a table to say where the backing space for each page is
located.
- This corresponds to the page table used to tell where in
real memory a page is located.
- The format of the ``memory page table'' is determined by
the hardware since the hardware modifies/accesses it.
- The format of the ``disk page table'' is decided by the OS
designers and is machine independent.
- If the format of the memory page table was flexible, then
we might well keep the disk information in it as well.
4.7.6: Separation of Policy and Mechanism
Skipped.
4.8: Segmentation
Up to now, the virtual address space has been contiguous.
- Among other issues this makes memory management difficult when
there are more that two dynamically growing regions.
- With two regions you start them on opposite sides of the virtual
space as we did before.
- Better is to have many virtual address spaces each starting at
zero.
- This split up is user visible.
- Without segmentation (equivalently said with just one segment) all
procedures are packed together so if one changes in size all the virtual
addresses following are changed and the program must be re-linked.
- Eases flexible protection and sharing (share a segment). For
example, can have a shared library.
Homework: 37.
** Two Segments
Late PDP-10s and TOPS-10
- One shared text segment, that can also contain shared
(normally read only) data.
- One (private) writable data segment.
- Permission bits on each segment.
- Which kind of segment is better to evict?
- Swap out shared segment hurts many tasks.
- The shared segment is read only (probably) so no writeback
is needed.
- ``One segment'' is OS/MVT done above.
** Three Segments
Traditional (early) Unix shown at right.
- Shared text marked execute only.
- Data segment (global and static variables).
- Stack segment (automatic variables).
- (In reality, since the text doesn't grow, this was sometimes
treated as 2 segments.)
** Four Segments
Just kidding.