Operating Systems
Chapter 5: Input/Output
5.1: Principles of I/O Hardware
5.1.1: I/O Devices
- Not much to say. Devices are varied.
- Block versus character devices:
- Devices, such as disks and CDROMs, with addressable chunks
(sectors in this case) are called block
devices,
These devices support seeking.
- Devices, such as Ethernet and modem connections, that are a
stream of characters are called character
devices.
These devices do not support seeking.
- Some cases, like tapes, are not so clear.
5.1.2: Device Controllers
These are the ``real devices'' as far as the OS is concerned. That
is the OS code is written with the controller spec in hand not with
the device spec.
The figure in the book is so oversimplified as to be borderline
false. The following picture is closer to the truth (but really there
are several I/O buses of different speeds).
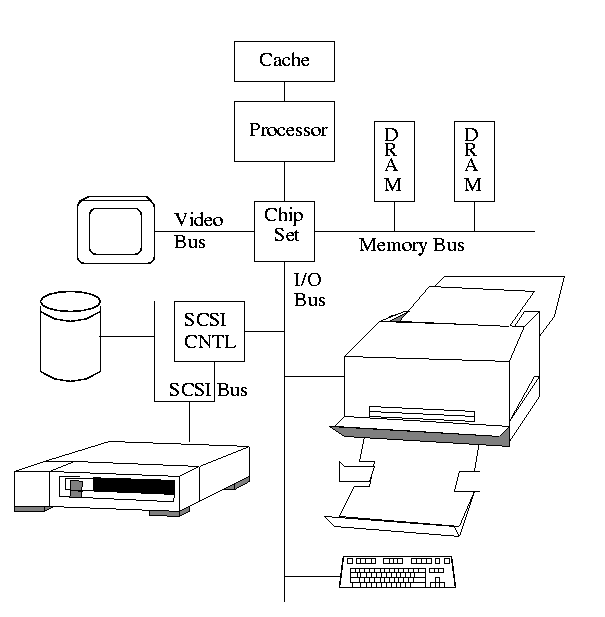
- The controller abstracts away some of the low level features of
the device.
- For disks, the controller does error checking, buffering and
handles interleaving of sectors. (Sectors are interleaved if the
controller or CPU cannot handle the data rate and would otherwise have
to wait a full revolution. This is not a concern with modern systems
since the electronics have increased in speed faster than the
devices.)
- For analog monitors (CRTs) the controller does
a great deal. Analog video is far from a bunch of ones and
zeros.
- Controllers are also called adaptors.
Using a controller
Think of a disk controller and a read request. The goal is to copy
data from the disk to some portion of the central memory. How do we
do this?
- The controller contains a microprocessor and memory and is
connected to the disk (by a cable).
- When the controller asks the disk to read a sector, the contents
come to the controller via the cable and are stored by the controller
in its memory.
- The question is how does the OS, which is running on another
processor, let the controller know that a disk read is desired and how
is the data eventually moved from the controllers memory to the
general system memory.
- Typically the interface the OS sees consists of some device
registers located on the controller.
- These are memory locations into which the OS writes
information such as sector to access, read vs. write, length,
where in system memory to put the data (for a read) or from where
to take the data (for a write).
- There is also typically a device register that acts as a
``go button''.
- There are also devices registers that the OS reads, such as
status of the controller, errors found, etc.
- So now the question is how does the OS read and write the device
register.
- With Memory-mapped I/O the device registers
appear as normal memory. All that is needed is to know at which
address each device regester appears. Then the OS uses normal
load and store instructions to write the registers.
- Some systems instead have a special ``I/O space'' into which
the registers are mapped and require the use of special I/O
instructions to accomplish the load and store. From a conceptual
point of view there is no difference between the two models.
Homework: 2
5.1.3: Direct Memory Access (DMA)
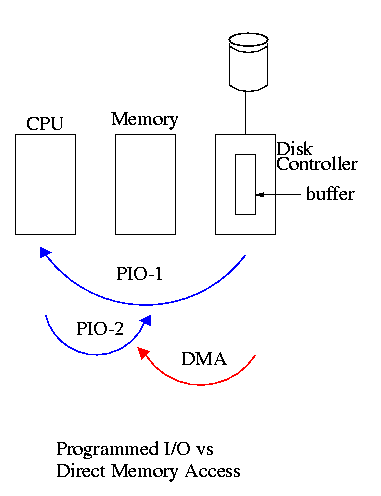
- With or without DMA, the disk controller pulls the desired data
from the disk to its buffer (and pushes data from the buffer to the
disk).
- Without DMA, i.e., with programmed I/O (PIO), the
cpu then does loads and stores (or I/O instructions) to copy the data
from the buffer to the desired memory location.
- With a DMA controller, the controller writes the memory without
intervention of the CPU.
- Clearly DMA saves CPU work. But this might not be important if
the CPU is limited by the memory or by system buses.
- Very important is that there is less data movement so the buses
are used less and the entire operation takes less time.
- Since PIO is pure software it is easier to change, which is an
advantage.
- DMA does need a number of bus transfers from the CPU to the
controller to specify the DMA. So DMA is most effective for large
transfers where the setup is amortized.
- Why have the buffer? Why not just go from the disk straight to
the memory.
Answer: Speed matching. The disk supplies data at a fixed rate, which might
exceed the rate the memory can accept it. In particular the memory
might be busy servicing a request from the processor or from another
DMA controller.
Homework: 5
================ Start Lecture #12
================
Note:
I improved the presentation of disk controllers given last time
(unfortunately, this was very easy to do). In particular, I suggest
you read the new small section entitled ``Using a controller''. Both
the giant page and the page for lecture-11 now include this new
section.
5.2: Principles of I/O Software
As with any large software system, good design and layering is
important.
5.2.1: Goals of the I/O Software
Device independence
We want to have most of the OS, unaware of the characteristics of
the specific devices attached to the system. Indeed we also want the
OS to be largely unaware of the CPU type itself.
Due to this device independence, programs are
written to read and write generic devices and then at run time
specific devices are assigned. Writing to a disk has differences from
writing to a terminal, but Unix cp and DOS copy do not see these
differences. Indeed, most of the OS, including the file system code,
is unaware of whether the device is a floppy or hard disk.
Uniform naming
Recall that we discussed the value
of the name space implemented by file systems. There is no dependence
between the name of the file and the device on which it is stored. So
a file called IAmStoredOnAHardDisk might well be stored on a floppy disk.
Error handling
There are several aspects to error handling including: detection,
correction (if possible) and reporting.
- Detection should be done as close to where the error occurred as
possible before more damage is done (fault containment). This is not
trivial.
- Correction is sometimes easy, for example ECC memory does this
automatically (but the OS wants to know about the error so that it can
schedule replacement of the faulty chips before unrecoverable double
errors occur).
Other easy cases include successful retries for failed ethernet
transmissions. In this example, while logging is appropriate, it is
quite reasonable for no action to be taken.
- Error reporting tends to be awful. The trouble is that the error
occurs at a low level but by the time it is reported the
context is lost. Unix/Linux in particular is horrible in this area.
Creating the illusion of synchronous I/O
- I/O must be asynchronous for good performance. That is
the OS cannot simply wait for an I/O to complete. Instead, it
proceeds with other activities and responds to the notification when
the I/O has finished.
- Users (mostly) want no part of this. The code sequence
Read X
Y <-- X+1
Print Y
should print a value one greater than that read. But if the
assignment is performed before the read completes, the wrong value is
assigned.
- Performance junkies sometimes do want the asynchrony so that they
can have another portion of their program executed while the I/O is
underway. That is they implement a mini-scheduler in their
application code.
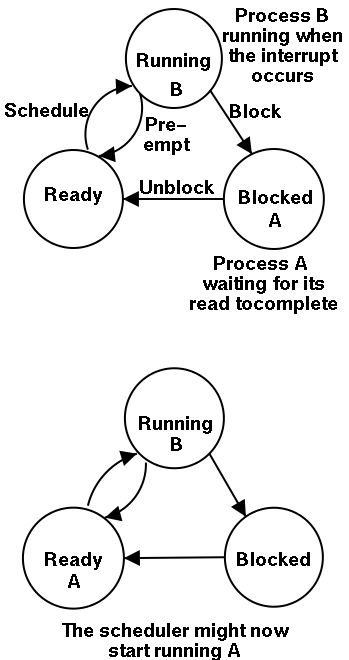
Sharable vs dedicated devices
For devices like printers and tape drives, only one user at a time
is permitted. These are called serially reusable
devices, and are studied next chapter.
Devices like disks and Ethernet ports can be shared by processes
running concurrently.
Layering
Layers of abstraction as usual prove to be effective. Most systems
are believed to use the following layers (but for many systems, the OS
code is not available for inspection).
- User level I/O routines.
- Device independent I/O software.
- Device drivers.
- Interrupt handlers.
We give a bottom up explanation.
5.2.2: Interrupt Handlers
We discussed an interrupt handler before when studying page faults.
Then it was called ``assembly language code''.
In the present case, we have a process blocked on I/O and the I/O
event has just completed. So the goal is to make the process ready.
Possible methods are.
- Releasing a semaphore on which the process is waiting.
- Sending a message to the process.
- Inserting the process table entry onto the ready list.
Once the process is ready, it is up to the scheduler to decide when
it should run.
5.2.3: Device Drivers
The portion of the OS that ``knows'' the characteristics of the
controller.
The driver has two ``parts'' corresponding to its two access
points. Recall the following figure from the beginning of the course.
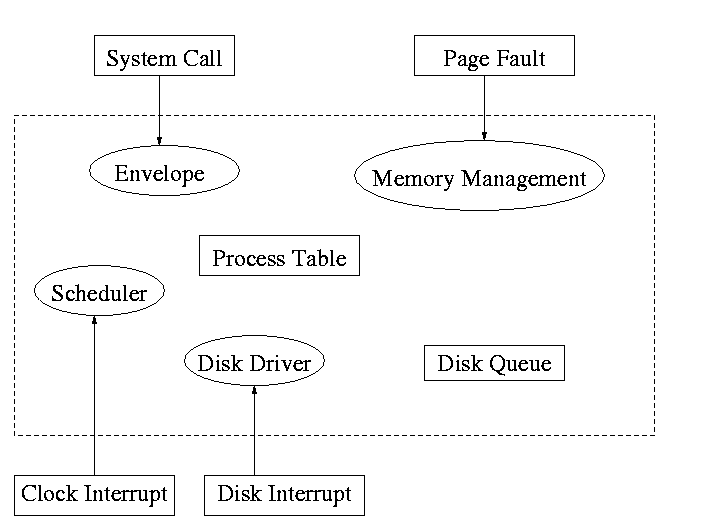
- Accessed by the main line OS via the envelope in response to an I/O
system call. The portion of the driver accessed in this way is
sometimes call the ``top'' part.
- Accessed by the interrupt handler when the I/O completes (this
completion is signaled by an interrupt). The portion of the driver
accessed in this way is sometimes call the ``bottom'' part.
Tanenbaum describes the actions of the driver assuming it is
implemented as a process (which he recommends). I give both that view
point and the self-service paradigm in which the driver is invoked by
the OS acting in behalf of a user process (more precisely the process
shifts into kernel mode).
Driver in a self-service paradigm
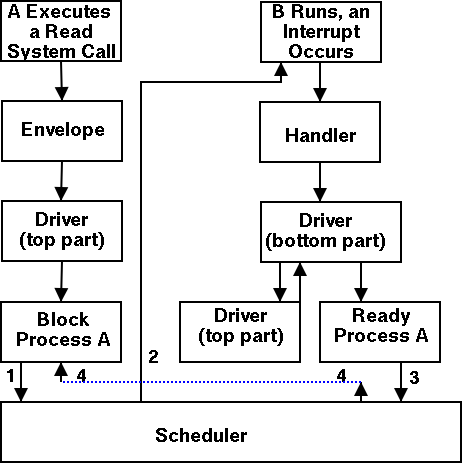
- The user (A) issues an I/O system call.
- The main line, machine independent, OS prepares a
generic request for the driver and calls (the top part of)
the driver.
- If the driver was idle (i.e., the controller was idle), the
driver writes device registers on the controller ending with a
command for the controller to begin the actual I/O.
- If the controller was busy (doing work the driver gave it
previously), the driver simply queues the current request (the
driver dequeues this request below).
- The driver jumps to the scheduler indicating that the current
process should be blocked.
- The scheduler blocks A and runs (say) B.
- B starts running.
- An interrupt arrives (i.e., an I/O has been completed).
- The interrupt handler invokes (the bottom part of) the driver.
- The driver informs the main line perhaps passing data and
surely passing status (error, OK).
- The top part is called to start another I/O if the queue is
nonempty. We know the controller is free. Why?
Answer: We just received an interrupt saying so.
- The driver jumps to the scheduler indicating that process A should
be made ready.
- The scheduler picks a ready process to run. Assume it picks A.
- A resumes in the driver, which returns to the main line, which
returns to the user code.
Driver as a process (Tanenbaum) (less detailed than above)
- The user issues an I/O request. The main line OS prepares a
generic request (e.g. read, not read using Buslogic BT-958 SCSI controller)
for the driver and the driver is awakened (perhaps a message is sent to
the driver to do both jobs).
- The driver wakes up.
- If the driver was idle (i.e., the controller is idle), the
driver writes device registers on the controller ending with a
command for the controller to begin the actual I/O.
- If the controller is busy (doing work the driver gave it), the
driver simply queues the current request (the driver dequeues this
below).
- The driver blocks waiting for an interrupt or for more
requests.
- An interrupt arrives (i.e., an I/O has been completed).
- The driver wakes up.
- The driver informs the main line perhaps passing data and
surely passing status (error, OK).
- The driver finds the next work item or blocks.
- If the queue of requests is non-empty, dequeue one and
proceed as if just received a request from the main line.
- If queue is empty, the driver blocks waiting for an
interrupt or a request from the main line.
5.2.4: Device-Independent I/O Software
The device-independent code does most of the functionality, but not
necessarily most of the code since there can be many drivers
all doing essentially the same thing in slightly different ways due to
slightly different controllers.
- Naming. Again an important O/S functionality.
Must offer a consistent interface to the device drivers.
- In Unix this is done by associating each device with a
(special) file in the /dev directory.
- The inodes for these files contain an indication that these
are special files and also contain so called major and minor
device numbers.
- The major device number gives the number of the driver.
(These numbers are rather ad hoc, they correspond to the position
of the function pointer to the driver in a table of function
pointers.)
- The minor number indicates for which device (e.g., which scsi
cdrom drive) the request is intended
- Protection. A wide range of possibilities are
actually done in real systems. Including both extreme examples of
everything is permitted and nothing is permitted (directly).
- In ms-dos any process can write to any file. Presumably, our
offensive nuclear missile launchers do not run dos.
- In IBM and other mainframe OS's, normal processors do not
access devices. Indeed the main CPU doesn't issue the I/O
requests. Instead an I/O channel is used and the mainline
constructs a channel program and tells the channel to invoke it.
- Unix uses normal rwx bits on files in /dev (I don't believe x
is used).
- Buffering is necessary since requests come in a
size specified by the user and data is delivered in a size specified
by the device.
- Enforce exclusive access for non-shared devices
like tapes.
5.2.5: User-Space Software
A good deal of I/O code is actually executed in user space. Some
is in library routines linked into user programs and some is in daemon
processes.
- Some library routines are trivial and just move their arguments
into the correct place (e.g., a specific register) and then issue a
trap to the correct system call to do the real work.
- Some, notably standard I/O (stdio) in Unix, are definitely not
trivial. For example consider the formatting of floating point
numbers done in printf and the reverse operation done in scanf.
- Printing to a local printer is often performed in part by a
regular program (lpr in Unix) and part by a daemon
(lpd in Unix).
The daemon might be started when the system boots or might be started
on demand. I guess it is called a daemon because it is not under the
control of any user. Does anyone know the
reason.
- Printing uses spooling, i.e., the file to be
printed is copied somewhere by lpr and then the daemon works with this
copy. Mail uses a similar technique (but generally it is called
queuing, not spooling).
Homework: 6, 7, 8.
5.3: Disks
The ideal storage device is
- Fast
- Big (in capacity)
- Cheap
- Impossible
Disks are big and cheap, but slow.
5.3.1: Disk Hardware
Show a real disk opened up and illustrate the components
- Platter
- Surface
- Head
- Track
- Sector
- Cylinder
- Seek time
- Rotational latency
- Transfer time
Overlapping I/O operations is important. Many controllers can do
overlapped seeks, i.e. issue a seek to one disk while another is
already seeking.
Despite what Tanenbaum says, modern disks cheat and do not have
the same number of sectors on outer cylinders as on inner one. Often
the controller ``cover for them'' and protect the lie.
Again contrary to Tanenbaum, it is not true that when one head is
reading from cylinder C, all the heads can read from cylinder C with
no penalty.
Choice of block size
- We discussed this before when studying page size.
- Current commodity disk characteristics (not for laptops) result in
about 15ms to transfer the first byte and 10K bytes per ms for
subsequent bytes (if contiguous).
- Rotation rate is 5400, 7600, or 10,000 RPM (15K just now
available).
- Recall that 6000 RPM is 100 rev/sec or one rev
per 10ms. So half a rev (the average time for to rotate to a
given point) is 5ms.
- Transfer rates around 10MB/sec = 10KB/ms.
- Seek time around 10ms.
- This favors large blocks, 100KB or more.
- But the internal fragmentation would be severe since many files
are small.
- Multiple block sizes have been tried as have techniques to try to
have consecutive blocks of a given file near each other.
- Typical block sizes are 4KB-8KB.
5.3.2: Disk Arm Scheduling Algorithms
These algorithms are relevant only if there are several I/O
requests pending. For many PCs this is not the case. For most
commercial applications, I/O is crucial.
- FCFS (First Come First Served): Simple but has long delays.
- Pick: Same as FCFS but pick up requests for cylinders that are
passed on the way to the next FCFS request.
- SSTF (Shortest Seek Time First): Greedy algorithm. Can starve
requests for outer cylinders and almost always favors middle requests.
- Scan (Look, Elevator): The method used by an old fashioned
jukebox (remember ``Happy Days'') and by elevators. The disk arm
proceeds in one direction picking up all requests until there are no
more requests in this direction at which point it goes back the other
direction. This favors requests in the middle, but can't starve any
requests.
- C-Scan (C-look, Circular Scan/Look): Similar to Scan but only
service requests when moving in one direction. When going in the
other direction, go directly to the furthest away request. This
doesn't favor any spot on the disk. Indeed, it treats the cylinders
as though they were a clock, i.e. after the highest numbered cylinder
comes cylinder 0.
- N-step Scan: This is what the natural implementation of Scan
gives.
- While the disk is servicing a Scan direction, the controller
gathers up new requests and sorts them.
- At the end of the current sweep, the new list becomes the next
sweep.
Minimizing Rotational Latency
Use Scan, which is the same as C-Scan. Why?
Because the disk only rotates in one direction.
Homework: 9, 10.
================ Start Lecture #13
================
RAID (Redundant Array of Inexpensive Disks)
- Tanenbaum's treatment is not very good.
- The name RAID is from Berkeley.
- IBM changed the name to Redundant Array of Independent
Disks. I wonder why?
- A simple form is mirroring, where two disks contain the
same data.
- Another simple form is striping (interleaving) where consecutive
blocks are spread across multiple disks. This helps bandwidth, but is
not redundant. Thus it shouldn't be called RAID, but it sometimes is.
- One of the normal RAID methods is to have N (say 4) data disks and one
parity disk. Data is striped across the data disks and the bitwise
parity of these sectors is written in the corresponding sector of the
parity disk.
- On a read if the block is bad (e.g., if the entire disk is bad or
even missing), the system automatically reads the other blocks in the
stripe and the parity block in the stripe. Then the missing block is
just the bitwise exclusive or of all these blocks.
- For reads this is very good. The failure free case has no penalty
(beyond the space overhead of the parity disk). The error case
requires N+1 (say 5) reads.
- A serious concern is the small write problem. Writing a sector
requires 4 I/O. Read the old data sector, compute the change, read
the parity, compute the new parity, write the new parity and the new
data sector. Hence one sector I/O became 4, which is a 300% penalty.
- Writing a full stripe is not bad. Compute the parity of the N
(say 4) data sectors to be written and then write the data sectors and
the parity sector. Thus 4 sector I/Os become 5, which is only a 20%
penalty and is smaller for larger N, i.e., larger stripes.
- A variation is to rotate the parity. That is, for some stripes
disk 1 has the parity, for others disk 2, etc. The purpose is to not
have a single parity disk since that disk is needed for all small
writes and could become a point of contention.
5.3.3: Error Handling
Disks error rates have dropped in recent years. Moreover, bad
block forwarding is done by the controller (or disk electronic) so
this topic is no longer as important for OS.
5.3.4: Track Caching
Often the disk/controller caches a track, since the
seek penalty has already been paid. In fact modern disks have
megabyte caches that hold recently read blocks. Since modern disks
cheat and don't have the same number of blocks on each track, it is
better for the disk electronics (and not the OS or controller) to do
the caching since it is the only part of the system to know the true
geometry.
5.3.5: Ram Disks
- Fairly clear. Organize a region of memory as a set of blocks and
pretend it is a disk.
- A problem is that memory is volatile.
- Often used during OS installation, before disk drivers are
available (there are many types of disk but all memory looks
the same so only one ram disk driver is needed).
5.4: Clocks
Also called timers.
5.4.1: Clock Hardware
- Generates an interrupt when timer goes to zero
- Counter reload can be automatic or under software (OS) control.
- If done automatically, the interrupt occurs periodically and thus
is perfect for generating a clock interrupt at a fixed period.
5.4.2: Clock Software
- TOD: Bump a counter each tick (clock interupt). If counter is
only 32 bits must worry about overflow so keep two counters: low order
and high order.
- Time quantum for RR: Decrement a counter at each tick. The quantum
expires when counter is zero. Load this counter when the scheduler
runs a process.
- Accounting: At each tick, bump a counter in the process table
entry for the currently running process.
- Alarm system call and system alarms:
- Users can request an alarm at some future time and the system
also needs to do things specific future times (e.g. turn off
floppy motor).
- The conceptually simplest solution is to have one timer for
each event. Instead, we simulate many timers with just one.
- The data structure on the right works well.
- The time in each list entry is the time after the
preceding entry that this entry's alarm is to ring. For example,
if the time is zero, this event occurs at the same time as the
previous event. The other
entry is a pointer to the action to perform.
- At each tick, decrement next-signal.
- When next-signal goes to zero,
process the first entry on the list and any others following
immediately after with a time of zero (which means they are to be
simultaneous with this alarm). Then set next-signal to the value
in the next alarm.
- Profiling
- Want a histogram giving how much time was spent in each 1KB
(say) block of code.
- At each tick check the PC and bump the appropriate counter.
- At the end of the run can assign the 1K blocks to software
modules.
- If use fine granularity (say 10B instead of 1KB) get higher
accuracy but more memory overhead.
Homework: 12
5.5: Terminals
5.5.1: Terminal Hardware
Quite dated. It is true that modern systems can communicate to a
hardwired ascii terminal, but most don't. Serial ports are used, but
they are normally connected to modems and then some protocol (SLIP,
PPP) is used not just a stream of ascii characters.
5.5.2: Memory-Mapped Terminals
- Less dated. But it still discusses the character not graphics
interface.
- Today, the idea is to have the software write into video memory
the bits to be put on the screen and then the graphics controller
converts these bits to analog signals for the monitor (actually laptop
displays and very modern monitors are digital).
- But it is much more complicated than this. The graphics
controllers can do a great deal of video themselves (like filling).
- This is a subject that would take many lectures to do well.
Keyboards
Tanenbaum description of keyboards is correct.
- At each key press and key release a code is written into the
keyboard controller and the computer is interrupted.
- By remembering which keys have been depressed and not released
the software can determine Cntl-A, Shift-B, etc.
5.5.3: Input Software
- We are just looking at keyboard input. Once again graphics is too
involved to be treated here.
- There are two fundamental modes of input, sometimes called
raw and cooked.
- In raw mode the application sees every ``character'' the user
types. Indeed, raw mode is character oriented.
- All the OS does is convert the keyboard ``scan codes'' to
``characters'' and and pass these characters to the application.
- Some examples
- down-cntl down-x up-x up-cntl is converted to cntl-x
- down-cntl up-cntl down-x up-x is converted to x
- down-cntl down-x up-cntl up-x is converted to cntl-x (I just
tried it to be sure).
- down-x down-cntl up-x up-cntl is converted to x
- Full screen editors use this mode.
- Cooked mode is line oriented. The OS delivers lines to the
application program.
- Special characters are interpreted as editing characters
(erase-previous-character, erase-previous-word, kill-line, etc).
- Erased characters are not seen by the application but are
erased by the keyboard driver.
- Need an escape character so that the editing characters can be
passed to the application if desired.
- The cooked characters must be echoed (what should one do if the
application is also generating output at this time?)
- The (possibly cooked) characters must be buffered until the
application issues a read (and an end-of-line EOL has been received
for cooked mode).
5.5.4: Output Software
Again too dated and the truth is too complicated to deal with in a
few minutes.
Homework: 16.
Allan Gottlieb